Why is choosing a suitable thermodynamic potential important?Using 'Euler's Homogeneous Function Theorem' to Justify Thermodynamic DerivationsI still don't understand how a gas with an equation of state f(T,p,V)=0 can change if 2 state functions are fixed?Spontaneity / Free Energy of Non-Isothermal ProcessWhy does this derivation of the dependence of free energy on pressure not work?Showing $mu_1=mu_2$ by minimization of thermodynamic potentialsIntuitive understanding and physical significance of Exergy, Gibbs free energy and differencesWhy is it the Helmholtz free energy that is minimised in the Ising model?An attempt at justifying effects of minima for Gibbs free energy and Helmholtz free energyDiffering definitions of Gibbs free energy and Helmholtz free energyWhat are 'external parameters' in Thermodynamics?
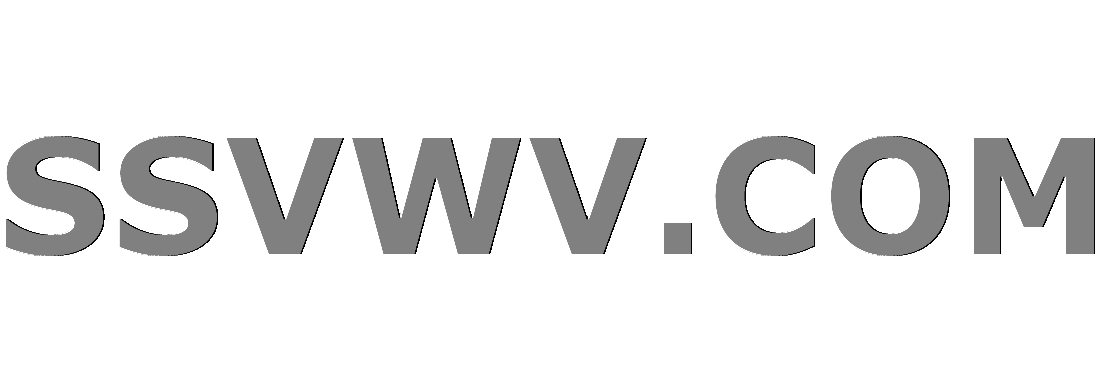
Multi tool use
Is there a way to save this session?
Are there regional foods in Westeros?
Is a hash a zero-knowledge proof?
Racetrack designers, assemble!
How to decline physical affection from a child whose parents are pressuring them?
Select row of data if next row contains zero
Asking for something with different prices
What is the most important characteristic of New Weird as a genre?
Why does my electric oven present the option of 40A and 50A breakers?
What should I do about a religious player who refuses to accept the existence of multiple gods in D&D?
Looking after a wayward brother in mother's will
What is a simple, physical situation where complex numbers emerge naturally?
Why does the UK have more political parties than the US?
Singlequote and backslash
Future enhancements for the finite element method
What people are called "кабан" and why?
Is it possible to kill all life on Earth?
Can a helicopter mask itself from Radar?
What's the most polite way to tell a manager "shut up and let me work"?
Creating Fictional Slavic Place Names
arcpy.GetParameterAsText not passing arguments to script?
How can I grammatically understand "Wir über uns"?
How do I truncate a csv file?
Why use water tanks from a retired Space Shuttle?
Why is choosing a suitable thermodynamic potential important?
Using 'Euler's Homogeneous Function Theorem' to Justify Thermodynamic DerivationsI still don't understand how a gas with an equation of state f(T,p,V)=0 can change if 2 state functions are fixed?Spontaneity / Free Energy of Non-Isothermal ProcessWhy does this derivation of the dependence of free energy on pressure not work?Showing $mu_1=mu_2$ by minimization of thermodynamic potentialsIntuitive understanding and physical significance of Exergy, Gibbs free energy and differencesWhy is it the Helmholtz free energy that is minimised in the Ising model?An attempt at justifying effects of minima for Gibbs free energy and Helmholtz free energyDiffering definitions of Gibbs free energy and Helmholtz free energyWhat are 'external parameters' in Thermodynamics?
$begingroup$
Say we are undergoing an isothermal process that eventually settles at equilibrium. $F$ will minimize at equilibrium, and if the entropy of the system is not held constant $U$ will not. Why do we care though? What do we afford to lose if we use $U$ anyway? What use do we have for $F$ if it has this property for isothermal processes?
Gibbs free energy minimizes only at constant pressure and temperature, so to me it sounds like a less general version of Helmholtz free energy. So why is it used?
thermodynamics potential
$endgroup$
add a comment |
$begingroup$
Say we are undergoing an isothermal process that eventually settles at equilibrium. $F$ will minimize at equilibrium, and if the entropy of the system is not held constant $U$ will not. Why do we care though? What do we afford to lose if we use $U$ anyway? What use do we have for $F$ if it has this property for isothermal processes?
Gibbs free energy minimizes only at constant pressure and temperature, so to me it sounds like a less general version of Helmholtz free energy. So why is it used?
thermodynamics potential
$endgroup$
$begingroup$
what do you mean by what do we care? If you use the energy instead of the Free-energy for an isothermal process then it is incorrect.
$endgroup$
– lakehal
May 16 at 19:26
1
$begingroup$
Incorrect how? I've never actually used the free energies before, and only know them in terms of how they're defined so that's why it's not clear to me.
$endgroup$
– sangstar
May 16 at 19:27
1
$begingroup$
@lakehal What are you talking about? There is no rule against "using energy instead of free-energy" for an isothermal process, you simply need to take account for the constant temperature in the rest of your analysis.
$endgroup$
– Drew
May 16 at 20:52
$begingroup$
@Drew but it is not minimised.
$endgroup$
– lakehal
May 16 at 21:25
add a comment |
$begingroup$
Say we are undergoing an isothermal process that eventually settles at equilibrium. $F$ will minimize at equilibrium, and if the entropy of the system is not held constant $U$ will not. Why do we care though? What do we afford to lose if we use $U$ anyway? What use do we have for $F$ if it has this property for isothermal processes?
Gibbs free energy minimizes only at constant pressure and temperature, so to me it sounds like a less general version of Helmholtz free energy. So why is it used?
thermodynamics potential
$endgroup$
Say we are undergoing an isothermal process that eventually settles at equilibrium. $F$ will minimize at equilibrium, and if the entropy of the system is not held constant $U$ will not. Why do we care though? What do we afford to lose if we use $U$ anyway? What use do we have for $F$ if it has this property for isothermal processes?
Gibbs free energy minimizes only at constant pressure and temperature, so to me it sounds like a less general version of Helmholtz free energy. So why is it used?
thermodynamics potential
thermodynamics potential
asked May 16 at 19:12


sangstarsangstar
1,3851824
1,3851824
$begingroup$
what do you mean by what do we care? If you use the energy instead of the Free-energy for an isothermal process then it is incorrect.
$endgroup$
– lakehal
May 16 at 19:26
1
$begingroup$
Incorrect how? I've never actually used the free energies before, and only know them in terms of how they're defined so that's why it's not clear to me.
$endgroup$
– sangstar
May 16 at 19:27
1
$begingroup$
@lakehal What are you talking about? There is no rule against "using energy instead of free-energy" for an isothermal process, you simply need to take account for the constant temperature in the rest of your analysis.
$endgroup$
– Drew
May 16 at 20:52
$begingroup$
@Drew but it is not minimised.
$endgroup$
– lakehal
May 16 at 21:25
add a comment |
$begingroup$
what do you mean by what do we care? If you use the energy instead of the Free-energy for an isothermal process then it is incorrect.
$endgroup$
– lakehal
May 16 at 19:26
1
$begingroup$
Incorrect how? I've never actually used the free energies before, and only know them in terms of how they're defined so that's why it's not clear to me.
$endgroup$
– sangstar
May 16 at 19:27
1
$begingroup$
@lakehal What are you talking about? There is no rule against "using energy instead of free-energy" for an isothermal process, you simply need to take account for the constant temperature in the rest of your analysis.
$endgroup$
– Drew
May 16 at 20:52
$begingroup$
@Drew but it is not minimised.
$endgroup$
– lakehal
May 16 at 21:25
$begingroup$
what do you mean by what do we care? If you use the energy instead of the Free-energy for an isothermal process then it is incorrect.
$endgroup$
– lakehal
May 16 at 19:26
$begingroup$
what do you mean by what do we care? If you use the energy instead of the Free-energy for an isothermal process then it is incorrect.
$endgroup$
– lakehal
May 16 at 19:26
1
1
$begingroup$
Incorrect how? I've never actually used the free energies before, and only know them in terms of how they're defined so that's why it's not clear to me.
$endgroup$
– sangstar
May 16 at 19:27
$begingroup$
Incorrect how? I've never actually used the free energies before, and only know them in terms of how they're defined so that's why it's not clear to me.
$endgroup$
– sangstar
May 16 at 19:27
1
1
$begingroup$
@lakehal What are you talking about? There is no rule against "using energy instead of free-energy" for an isothermal process, you simply need to take account for the constant temperature in the rest of your analysis.
$endgroup$
– Drew
May 16 at 20:52
$begingroup$
@lakehal What are you talking about? There is no rule against "using energy instead of free-energy" for an isothermal process, you simply need to take account for the constant temperature in the rest of your analysis.
$endgroup$
– Drew
May 16 at 20:52
$begingroup$
@Drew but it is not minimised.
$endgroup$
– lakehal
May 16 at 21:25
$begingroup$
@Drew but it is not minimised.
$endgroup$
– lakehal
May 16 at 21:25
add a comment |
2 Answers
2
active
oldest
votes
$begingroup$
Foundations
In a closed system, the four combined laws of thermodynamics are as follows:
$$ dU = TdS - pdV $$
$$ dH = TdS + Vdp $$
$$ dA = -SdT - pdV $$
$$ dG = -SdT + Vdp $$
You can obtain these in one of two ways. You can start from the first law (the first equation above) and apply the definitions of enthalpy, Helmholz energy, and Gibbs energy. Alternatively, you can start from the postulates of $U(V,n)$ and $S(U,n)$ and work through Legendre transforms. The imperative appreciation here is that derivations of these equations is NOT based on statements about what kind of process is occurring. They are fundamental and absolute expressions.
The other imperative statement is that we always need two and only two parameters to define the exact mechanical, thermal, chemical, and therefore thermodynamic state of a closed system with a pure substance. It is a statement of the Gibbs phase rule $F_max = (C - R) - Pi_min + 2 = (1 - 0) - 1 + 2 = 2$. This establishes why the expansions below consider only two parameters and no more as requirements to set the thermodynamic criteria for equilibrium or spontaneity.
Equilibrium
At equilibrium, a system must show no change in any state property. By review of the combined laws
- A process that occurs at constant entropy and volume will show no change in $U$
- A process that occurs at constant entropy and pressure will show no change in $H$
- A process that occurs at constant temperature and volume will show no change in $A$
- A process that occurs at constant temperature and pressure will show no change in $G$
This establishes the four criteria for equilibrium of any process.
$Delta U = 0$ at constant $(S, V)$
$Delta H = 0$ at constant $(S, p)$
$Delta A = 0$ at constant $(T, V)$
$Delta G = 0$ at constant $(T, p)$
We live (mostly) in a constant temperature + pressure world. Therefore, to determine whether a system is or is not at equilibrium, we work with (engineer processes using) changes in the Gibbs energy more than any of the other thermodynamic state functions.
Spontaneity
A process that is occurring in one of the four realms and is not at equilibrium must have a change in the respective state function that itself is not zero. We apply a systematic analysis starting from the Clausius form of the second law (heat flows spontaneously from hot to cold) to establish the convention for $dU$ in spontaneous processes. The rest of the expressions follow from it.
$Delta U < 0$ at constant $(S, V)$
$Delta S > 0$ at constant $(U, V)$
$Delta H < 0$ at constant $(S, p)$
$Delta A < 0$ at constant $(T, V)$
$Delta G < 0$ at constant $(T, p)$
Utility
One or the other of the expressions above is never more (or less) "useful". The framework is always first to define what parameters are held constant as the process occurs. That step defines which state function is to be used to establish equilibrium or spontaneity.
The mechanical work of a reversible process is always $w = pm int p dV$ (I leave the sign convention here as a hot discussion point that IUPAC folks and engineers banter over). Other work may be obtained from a process when it is spontaneous in constant $T, V$ or constant $T, p$ space. This is how we relate changes in Helmholtz or Gibbs energy to "free" work of a process.
$endgroup$
add a comment |
$begingroup$
$U$ (or actually $S$) is only relevant for an isolated system. This is not the case, for example, when you keep the temperature constant, since then heat must flow from the environment to your system (or vice versa) for $T$ to remain fixed.
But let's say the system you are interested in is small compared to the environment. Keeping track after all the thermodynamics of the surroundings is difficult, so we want to somehow treat the system alone without having to think all the time on what is happening outside. The way to do it, in the case of thermal contact only, is to define the Helmholtz free energy
$$F=U-TS$$
Here $U$ is the energy of the system, $S$ is its entropy but $T$ is the temperature of the environment (and it is fixed since your system is so small so that practically it has no effect on it). When $F$ is minimized, it can be shown (see here) that the entropy of the system + the environment is maximized, which is the condition you would expect for a system in thermal equilibrium.
Similarly, if your system can only exchange volume with the environment, the quantity that is minimized is known at the enthalpy
$$H=U+PV$$
In the case of both heat and volume exchange, you would use the Gibbs free energy
$$G=U-TS+PV$$
There is also a mathematical-inclined argument for this. You know that
$$rm dF=-Srm dT-Prm dV$$
so the natural variables of $F$ are $T$ and $V$, i.e. $F=Fleft(T,Vright)$. It means that $F$ is the right function to use if you can control both $T$ and $V$, which is the case for a system of constant volume in contact with a thermal bath. In the other cases
$$rm dH=Trm dS+Vrm dPLongrightarrow H=Hleft(S,Pright)$$
and
$$rm dG=-Srm dT+Vrm dPLongrightarrow G=Gleft(T,Pright)$$
You can clearly see which variables you can control and consequently which situations each thermodynamic potential fits. This mathematical trick of changing the variables of your function is known as the Legendre transformation. This is exactly the same as the relation between Lagrangians and Hamiltonians in classical mechanics.
To finish with an example, when treating liquid-gas phase transitions, it is customary to use the Gibbs free energy. This is the right thermodynamic potential since your system can exchange both its entropy $S$ and volume $V$ with the outside, such that the variables you control (the environment) are the corresponding conjugate variables - the temperature $T$ and the pressure $P$ respectively.
$endgroup$
$begingroup$
So $F$ is $U$ disregarding the effects of heat transferred to it by the environment? So what energy left over is the 'free' energy? And the other potentials are $U$ but accounting for the effects the environment will have on its internal energy?
$endgroup$
– sangstar
May 16 at 19:37
$begingroup$
Yes, and one can show that for a reversible process $W=-Delta F$, which is why $F$ is the free energy - what you can actually put into work.
$endgroup$
– eranreches
May 16 at 19:43
$begingroup$
Why when I've worked out the work done due to isothermal processes in the past, I was fine to work with internal energy? Shouldn't I be working in Helmholtz free energy since processes are isothermal?
$endgroup$
– sangstar
May 16 at 20:50
$begingroup$
To be pedantic, the IUPAC convections on the names for Helmholtz and Gibbs energies do not include the word "free", and the Helmholtz energy is $A$ not $F$.
$endgroup$
– Jeffrey J Weimer
May 16 at 20:53
$begingroup$
@sangstar The relation $rm dU=Trm dS-Prm dV$ where $rm dW=Prm dV$ is still valid, so you can indeed use $U$ to get the work. But note: the change is energy is not simply the work as in mechanics, but contains another term due to entropy exchange with the surroundings. The change in $F$ contains just the work for reversible process. In addition, $U$ is not minimized so $rm dU$ is non-zero in equilibrium.
$endgroup$
– eranreches
May 16 at 21:13
add a comment |
Your Answer
StackExchange.ready(function()
var channelOptions =
tags: "".split(" "),
id: "151"
;
initTagRenderer("".split(" "), "".split(" "), channelOptions);
StackExchange.using("externalEditor", function()
// Have to fire editor after snippets, if snippets enabled
if (StackExchange.settings.snippets.snippetsEnabled)
StackExchange.using("snippets", function()
createEditor();
);
else
createEditor();
);
function createEditor()
StackExchange.prepareEditor(
heartbeatType: 'answer',
autoActivateHeartbeat: false,
convertImagesToLinks: false,
noModals: true,
showLowRepImageUploadWarning: true,
reputationToPostImages: null,
bindNavPrevention: true,
postfix: "",
imageUploader:
brandingHtml: "Powered by u003ca class="icon-imgur-white" href="https://imgur.com/"u003eu003c/au003e",
contentPolicyHtml: "User contributions licensed under u003ca href="https://creativecommons.org/licenses/by-sa/3.0/"u003ecc by-sa 3.0 with attribution requiredu003c/au003e u003ca href="https://stackoverflow.com/legal/content-policy"u003e(content policy)u003c/au003e",
allowUrls: true
,
noCode: true, onDemand: true,
discardSelector: ".discard-answer"
,immediatelyShowMarkdownHelp:true
);
);
Sign up or log in
StackExchange.ready(function ()
StackExchange.helpers.onClickDraftSave('#login-link');
);
Sign up using Google
Sign up using Facebook
Sign up using Email and Password
Post as a guest
Required, but never shown
StackExchange.ready(
function ()
StackExchange.openid.initPostLogin('.new-post-login', 'https%3a%2f%2fphysics.stackexchange.com%2fquestions%2f480488%2fwhy-is-choosing-a-suitable-thermodynamic-potential-important%23new-answer', 'question_page');
);
Post as a guest
Required, but never shown
2 Answers
2
active
oldest
votes
2 Answers
2
active
oldest
votes
active
oldest
votes
active
oldest
votes
$begingroup$
Foundations
In a closed system, the four combined laws of thermodynamics are as follows:
$$ dU = TdS - pdV $$
$$ dH = TdS + Vdp $$
$$ dA = -SdT - pdV $$
$$ dG = -SdT + Vdp $$
You can obtain these in one of two ways. You can start from the first law (the first equation above) and apply the definitions of enthalpy, Helmholz energy, and Gibbs energy. Alternatively, you can start from the postulates of $U(V,n)$ and $S(U,n)$ and work through Legendre transforms. The imperative appreciation here is that derivations of these equations is NOT based on statements about what kind of process is occurring. They are fundamental and absolute expressions.
The other imperative statement is that we always need two and only two parameters to define the exact mechanical, thermal, chemical, and therefore thermodynamic state of a closed system with a pure substance. It is a statement of the Gibbs phase rule $F_max = (C - R) - Pi_min + 2 = (1 - 0) - 1 + 2 = 2$. This establishes why the expansions below consider only two parameters and no more as requirements to set the thermodynamic criteria for equilibrium or spontaneity.
Equilibrium
At equilibrium, a system must show no change in any state property. By review of the combined laws
- A process that occurs at constant entropy and volume will show no change in $U$
- A process that occurs at constant entropy and pressure will show no change in $H$
- A process that occurs at constant temperature and volume will show no change in $A$
- A process that occurs at constant temperature and pressure will show no change in $G$
This establishes the four criteria for equilibrium of any process.
$Delta U = 0$ at constant $(S, V)$
$Delta H = 0$ at constant $(S, p)$
$Delta A = 0$ at constant $(T, V)$
$Delta G = 0$ at constant $(T, p)$
We live (mostly) in a constant temperature + pressure world. Therefore, to determine whether a system is or is not at equilibrium, we work with (engineer processes using) changes in the Gibbs energy more than any of the other thermodynamic state functions.
Spontaneity
A process that is occurring in one of the four realms and is not at equilibrium must have a change in the respective state function that itself is not zero. We apply a systematic analysis starting from the Clausius form of the second law (heat flows spontaneously from hot to cold) to establish the convention for $dU$ in spontaneous processes. The rest of the expressions follow from it.
$Delta U < 0$ at constant $(S, V)$
$Delta S > 0$ at constant $(U, V)$
$Delta H < 0$ at constant $(S, p)$
$Delta A < 0$ at constant $(T, V)$
$Delta G < 0$ at constant $(T, p)$
Utility
One or the other of the expressions above is never more (or less) "useful". The framework is always first to define what parameters are held constant as the process occurs. That step defines which state function is to be used to establish equilibrium or spontaneity.
The mechanical work of a reversible process is always $w = pm int p dV$ (I leave the sign convention here as a hot discussion point that IUPAC folks and engineers banter over). Other work may be obtained from a process when it is spontaneous in constant $T, V$ or constant $T, p$ space. This is how we relate changes in Helmholtz or Gibbs energy to "free" work of a process.
$endgroup$
add a comment |
$begingroup$
Foundations
In a closed system, the four combined laws of thermodynamics are as follows:
$$ dU = TdS - pdV $$
$$ dH = TdS + Vdp $$
$$ dA = -SdT - pdV $$
$$ dG = -SdT + Vdp $$
You can obtain these in one of two ways. You can start from the first law (the first equation above) and apply the definitions of enthalpy, Helmholz energy, and Gibbs energy. Alternatively, you can start from the postulates of $U(V,n)$ and $S(U,n)$ and work through Legendre transforms. The imperative appreciation here is that derivations of these equations is NOT based on statements about what kind of process is occurring. They are fundamental and absolute expressions.
The other imperative statement is that we always need two and only two parameters to define the exact mechanical, thermal, chemical, and therefore thermodynamic state of a closed system with a pure substance. It is a statement of the Gibbs phase rule $F_max = (C - R) - Pi_min + 2 = (1 - 0) - 1 + 2 = 2$. This establishes why the expansions below consider only two parameters and no more as requirements to set the thermodynamic criteria for equilibrium or spontaneity.
Equilibrium
At equilibrium, a system must show no change in any state property. By review of the combined laws
- A process that occurs at constant entropy and volume will show no change in $U$
- A process that occurs at constant entropy and pressure will show no change in $H$
- A process that occurs at constant temperature and volume will show no change in $A$
- A process that occurs at constant temperature and pressure will show no change in $G$
This establishes the four criteria for equilibrium of any process.
$Delta U = 0$ at constant $(S, V)$
$Delta H = 0$ at constant $(S, p)$
$Delta A = 0$ at constant $(T, V)$
$Delta G = 0$ at constant $(T, p)$
We live (mostly) in a constant temperature + pressure world. Therefore, to determine whether a system is or is not at equilibrium, we work with (engineer processes using) changes in the Gibbs energy more than any of the other thermodynamic state functions.
Spontaneity
A process that is occurring in one of the four realms and is not at equilibrium must have a change in the respective state function that itself is not zero. We apply a systematic analysis starting from the Clausius form of the second law (heat flows spontaneously from hot to cold) to establish the convention for $dU$ in spontaneous processes. The rest of the expressions follow from it.
$Delta U < 0$ at constant $(S, V)$
$Delta S > 0$ at constant $(U, V)$
$Delta H < 0$ at constant $(S, p)$
$Delta A < 0$ at constant $(T, V)$
$Delta G < 0$ at constant $(T, p)$
Utility
One or the other of the expressions above is never more (or less) "useful". The framework is always first to define what parameters are held constant as the process occurs. That step defines which state function is to be used to establish equilibrium or spontaneity.
The mechanical work of a reversible process is always $w = pm int p dV$ (I leave the sign convention here as a hot discussion point that IUPAC folks and engineers banter over). Other work may be obtained from a process when it is spontaneous in constant $T, V$ or constant $T, p$ space. This is how we relate changes in Helmholtz or Gibbs energy to "free" work of a process.
$endgroup$
add a comment |
$begingroup$
Foundations
In a closed system, the four combined laws of thermodynamics are as follows:
$$ dU = TdS - pdV $$
$$ dH = TdS + Vdp $$
$$ dA = -SdT - pdV $$
$$ dG = -SdT + Vdp $$
You can obtain these in one of two ways. You can start from the first law (the first equation above) and apply the definitions of enthalpy, Helmholz energy, and Gibbs energy. Alternatively, you can start from the postulates of $U(V,n)$ and $S(U,n)$ and work through Legendre transforms. The imperative appreciation here is that derivations of these equations is NOT based on statements about what kind of process is occurring. They are fundamental and absolute expressions.
The other imperative statement is that we always need two and only two parameters to define the exact mechanical, thermal, chemical, and therefore thermodynamic state of a closed system with a pure substance. It is a statement of the Gibbs phase rule $F_max = (C - R) - Pi_min + 2 = (1 - 0) - 1 + 2 = 2$. This establishes why the expansions below consider only two parameters and no more as requirements to set the thermodynamic criteria for equilibrium or spontaneity.
Equilibrium
At equilibrium, a system must show no change in any state property. By review of the combined laws
- A process that occurs at constant entropy and volume will show no change in $U$
- A process that occurs at constant entropy and pressure will show no change in $H$
- A process that occurs at constant temperature and volume will show no change in $A$
- A process that occurs at constant temperature and pressure will show no change in $G$
This establishes the four criteria for equilibrium of any process.
$Delta U = 0$ at constant $(S, V)$
$Delta H = 0$ at constant $(S, p)$
$Delta A = 0$ at constant $(T, V)$
$Delta G = 0$ at constant $(T, p)$
We live (mostly) in a constant temperature + pressure world. Therefore, to determine whether a system is or is not at equilibrium, we work with (engineer processes using) changes in the Gibbs energy more than any of the other thermodynamic state functions.
Spontaneity
A process that is occurring in one of the four realms and is not at equilibrium must have a change in the respective state function that itself is not zero. We apply a systematic analysis starting from the Clausius form of the second law (heat flows spontaneously from hot to cold) to establish the convention for $dU$ in spontaneous processes. The rest of the expressions follow from it.
$Delta U < 0$ at constant $(S, V)$
$Delta S > 0$ at constant $(U, V)$
$Delta H < 0$ at constant $(S, p)$
$Delta A < 0$ at constant $(T, V)$
$Delta G < 0$ at constant $(T, p)$
Utility
One or the other of the expressions above is never more (or less) "useful". The framework is always first to define what parameters are held constant as the process occurs. That step defines which state function is to be used to establish equilibrium or spontaneity.
The mechanical work of a reversible process is always $w = pm int p dV$ (I leave the sign convention here as a hot discussion point that IUPAC folks and engineers banter over). Other work may be obtained from a process when it is spontaneous in constant $T, V$ or constant $T, p$ space. This is how we relate changes in Helmholtz or Gibbs energy to "free" work of a process.
$endgroup$
Foundations
In a closed system, the four combined laws of thermodynamics are as follows:
$$ dU = TdS - pdV $$
$$ dH = TdS + Vdp $$
$$ dA = -SdT - pdV $$
$$ dG = -SdT + Vdp $$
You can obtain these in one of two ways. You can start from the first law (the first equation above) and apply the definitions of enthalpy, Helmholz energy, and Gibbs energy. Alternatively, you can start from the postulates of $U(V,n)$ and $S(U,n)$ and work through Legendre transforms. The imperative appreciation here is that derivations of these equations is NOT based on statements about what kind of process is occurring. They are fundamental and absolute expressions.
The other imperative statement is that we always need two and only two parameters to define the exact mechanical, thermal, chemical, and therefore thermodynamic state of a closed system with a pure substance. It is a statement of the Gibbs phase rule $F_max = (C - R) - Pi_min + 2 = (1 - 0) - 1 + 2 = 2$. This establishes why the expansions below consider only two parameters and no more as requirements to set the thermodynamic criteria for equilibrium or spontaneity.
Equilibrium
At equilibrium, a system must show no change in any state property. By review of the combined laws
- A process that occurs at constant entropy and volume will show no change in $U$
- A process that occurs at constant entropy and pressure will show no change in $H$
- A process that occurs at constant temperature and volume will show no change in $A$
- A process that occurs at constant temperature and pressure will show no change in $G$
This establishes the four criteria for equilibrium of any process.
$Delta U = 0$ at constant $(S, V)$
$Delta H = 0$ at constant $(S, p)$
$Delta A = 0$ at constant $(T, V)$
$Delta G = 0$ at constant $(T, p)$
We live (mostly) in a constant temperature + pressure world. Therefore, to determine whether a system is or is not at equilibrium, we work with (engineer processes using) changes in the Gibbs energy more than any of the other thermodynamic state functions.
Spontaneity
A process that is occurring in one of the four realms and is not at equilibrium must have a change in the respective state function that itself is not zero. We apply a systematic analysis starting from the Clausius form of the second law (heat flows spontaneously from hot to cold) to establish the convention for $dU$ in spontaneous processes. The rest of the expressions follow from it.
$Delta U < 0$ at constant $(S, V)$
$Delta S > 0$ at constant $(U, V)$
$Delta H < 0$ at constant $(S, p)$
$Delta A < 0$ at constant $(T, V)$
$Delta G < 0$ at constant $(T, p)$
Utility
One or the other of the expressions above is never more (or less) "useful". The framework is always first to define what parameters are held constant as the process occurs. That step defines which state function is to be used to establish equilibrium or spontaneity.
The mechanical work of a reversible process is always $w = pm int p dV$ (I leave the sign convention here as a hot discussion point that IUPAC folks and engineers banter over). Other work may be obtained from a process when it is spontaneous in constant $T, V$ or constant $T, p$ space. This is how we relate changes in Helmholtz or Gibbs energy to "free" work of a process.
edited May 16 at 22:14
answered May 16 at 20:43


Jeffrey J WeimerJeffrey J Weimer
3506
3506
add a comment |
add a comment |
$begingroup$
$U$ (or actually $S$) is only relevant for an isolated system. This is not the case, for example, when you keep the temperature constant, since then heat must flow from the environment to your system (or vice versa) for $T$ to remain fixed.
But let's say the system you are interested in is small compared to the environment. Keeping track after all the thermodynamics of the surroundings is difficult, so we want to somehow treat the system alone without having to think all the time on what is happening outside. The way to do it, in the case of thermal contact only, is to define the Helmholtz free energy
$$F=U-TS$$
Here $U$ is the energy of the system, $S$ is its entropy but $T$ is the temperature of the environment (and it is fixed since your system is so small so that practically it has no effect on it). When $F$ is minimized, it can be shown (see here) that the entropy of the system + the environment is maximized, which is the condition you would expect for a system in thermal equilibrium.
Similarly, if your system can only exchange volume with the environment, the quantity that is minimized is known at the enthalpy
$$H=U+PV$$
In the case of both heat and volume exchange, you would use the Gibbs free energy
$$G=U-TS+PV$$
There is also a mathematical-inclined argument for this. You know that
$$rm dF=-Srm dT-Prm dV$$
so the natural variables of $F$ are $T$ and $V$, i.e. $F=Fleft(T,Vright)$. It means that $F$ is the right function to use if you can control both $T$ and $V$, which is the case for a system of constant volume in contact with a thermal bath. In the other cases
$$rm dH=Trm dS+Vrm dPLongrightarrow H=Hleft(S,Pright)$$
and
$$rm dG=-Srm dT+Vrm dPLongrightarrow G=Gleft(T,Pright)$$
You can clearly see which variables you can control and consequently which situations each thermodynamic potential fits. This mathematical trick of changing the variables of your function is known as the Legendre transformation. This is exactly the same as the relation between Lagrangians and Hamiltonians in classical mechanics.
To finish with an example, when treating liquid-gas phase transitions, it is customary to use the Gibbs free energy. This is the right thermodynamic potential since your system can exchange both its entropy $S$ and volume $V$ with the outside, such that the variables you control (the environment) are the corresponding conjugate variables - the temperature $T$ and the pressure $P$ respectively.
$endgroup$
$begingroup$
So $F$ is $U$ disregarding the effects of heat transferred to it by the environment? So what energy left over is the 'free' energy? And the other potentials are $U$ but accounting for the effects the environment will have on its internal energy?
$endgroup$
– sangstar
May 16 at 19:37
$begingroup$
Yes, and one can show that for a reversible process $W=-Delta F$, which is why $F$ is the free energy - what you can actually put into work.
$endgroup$
– eranreches
May 16 at 19:43
$begingroup$
Why when I've worked out the work done due to isothermal processes in the past, I was fine to work with internal energy? Shouldn't I be working in Helmholtz free energy since processes are isothermal?
$endgroup$
– sangstar
May 16 at 20:50
$begingroup$
To be pedantic, the IUPAC convections on the names for Helmholtz and Gibbs energies do not include the word "free", and the Helmholtz energy is $A$ not $F$.
$endgroup$
– Jeffrey J Weimer
May 16 at 20:53
$begingroup$
@sangstar The relation $rm dU=Trm dS-Prm dV$ where $rm dW=Prm dV$ is still valid, so you can indeed use $U$ to get the work. But note: the change is energy is not simply the work as in mechanics, but contains another term due to entropy exchange with the surroundings. The change in $F$ contains just the work for reversible process. In addition, $U$ is not minimized so $rm dU$ is non-zero in equilibrium.
$endgroup$
– eranreches
May 16 at 21:13
add a comment |
$begingroup$
$U$ (or actually $S$) is only relevant for an isolated system. This is not the case, for example, when you keep the temperature constant, since then heat must flow from the environment to your system (or vice versa) for $T$ to remain fixed.
But let's say the system you are interested in is small compared to the environment. Keeping track after all the thermodynamics of the surroundings is difficult, so we want to somehow treat the system alone without having to think all the time on what is happening outside. The way to do it, in the case of thermal contact only, is to define the Helmholtz free energy
$$F=U-TS$$
Here $U$ is the energy of the system, $S$ is its entropy but $T$ is the temperature of the environment (and it is fixed since your system is so small so that practically it has no effect on it). When $F$ is minimized, it can be shown (see here) that the entropy of the system + the environment is maximized, which is the condition you would expect for a system in thermal equilibrium.
Similarly, if your system can only exchange volume with the environment, the quantity that is minimized is known at the enthalpy
$$H=U+PV$$
In the case of both heat and volume exchange, you would use the Gibbs free energy
$$G=U-TS+PV$$
There is also a mathematical-inclined argument for this. You know that
$$rm dF=-Srm dT-Prm dV$$
so the natural variables of $F$ are $T$ and $V$, i.e. $F=Fleft(T,Vright)$. It means that $F$ is the right function to use if you can control both $T$ and $V$, which is the case for a system of constant volume in contact with a thermal bath. In the other cases
$$rm dH=Trm dS+Vrm dPLongrightarrow H=Hleft(S,Pright)$$
and
$$rm dG=-Srm dT+Vrm dPLongrightarrow G=Gleft(T,Pright)$$
You can clearly see which variables you can control and consequently which situations each thermodynamic potential fits. This mathematical trick of changing the variables of your function is known as the Legendre transformation. This is exactly the same as the relation between Lagrangians and Hamiltonians in classical mechanics.
To finish with an example, when treating liquid-gas phase transitions, it is customary to use the Gibbs free energy. This is the right thermodynamic potential since your system can exchange both its entropy $S$ and volume $V$ with the outside, such that the variables you control (the environment) are the corresponding conjugate variables - the temperature $T$ and the pressure $P$ respectively.
$endgroup$
$begingroup$
So $F$ is $U$ disregarding the effects of heat transferred to it by the environment? So what energy left over is the 'free' energy? And the other potentials are $U$ but accounting for the effects the environment will have on its internal energy?
$endgroup$
– sangstar
May 16 at 19:37
$begingroup$
Yes, and one can show that for a reversible process $W=-Delta F$, which is why $F$ is the free energy - what you can actually put into work.
$endgroup$
– eranreches
May 16 at 19:43
$begingroup$
Why when I've worked out the work done due to isothermal processes in the past, I was fine to work with internal energy? Shouldn't I be working in Helmholtz free energy since processes are isothermal?
$endgroup$
– sangstar
May 16 at 20:50
$begingroup$
To be pedantic, the IUPAC convections on the names for Helmholtz and Gibbs energies do not include the word "free", and the Helmholtz energy is $A$ not $F$.
$endgroup$
– Jeffrey J Weimer
May 16 at 20:53
$begingroup$
@sangstar The relation $rm dU=Trm dS-Prm dV$ where $rm dW=Prm dV$ is still valid, so you can indeed use $U$ to get the work. But note: the change is energy is not simply the work as in mechanics, but contains another term due to entropy exchange with the surroundings. The change in $F$ contains just the work for reversible process. In addition, $U$ is not minimized so $rm dU$ is non-zero in equilibrium.
$endgroup$
– eranreches
May 16 at 21:13
add a comment |
$begingroup$
$U$ (or actually $S$) is only relevant for an isolated system. This is not the case, for example, when you keep the temperature constant, since then heat must flow from the environment to your system (or vice versa) for $T$ to remain fixed.
But let's say the system you are interested in is small compared to the environment. Keeping track after all the thermodynamics of the surroundings is difficult, so we want to somehow treat the system alone without having to think all the time on what is happening outside. The way to do it, in the case of thermal contact only, is to define the Helmholtz free energy
$$F=U-TS$$
Here $U$ is the energy of the system, $S$ is its entropy but $T$ is the temperature of the environment (and it is fixed since your system is so small so that practically it has no effect on it). When $F$ is minimized, it can be shown (see here) that the entropy of the system + the environment is maximized, which is the condition you would expect for a system in thermal equilibrium.
Similarly, if your system can only exchange volume with the environment, the quantity that is minimized is known at the enthalpy
$$H=U+PV$$
In the case of both heat and volume exchange, you would use the Gibbs free energy
$$G=U-TS+PV$$
There is also a mathematical-inclined argument for this. You know that
$$rm dF=-Srm dT-Prm dV$$
so the natural variables of $F$ are $T$ and $V$, i.e. $F=Fleft(T,Vright)$. It means that $F$ is the right function to use if you can control both $T$ and $V$, which is the case for a system of constant volume in contact with a thermal bath. In the other cases
$$rm dH=Trm dS+Vrm dPLongrightarrow H=Hleft(S,Pright)$$
and
$$rm dG=-Srm dT+Vrm dPLongrightarrow G=Gleft(T,Pright)$$
You can clearly see which variables you can control and consequently which situations each thermodynamic potential fits. This mathematical trick of changing the variables of your function is known as the Legendre transformation. This is exactly the same as the relation between Lagrangians and Hamiltonians in classical mechanics.
To finish with an example, when treating liquid-gas phase transitions, it is customary to use the Gibbs free energy. This is the right thermodynamic potential since your system can exchange both its entropy $S$ and volume $V$ with the outside, such that the variables you control (the environment) are the corresponding conjugate variables - the temperature $T$ and the pressure $P$ respectively.
$endgroup$
$U$ (or actually $S$) is only relevant for an isolated system. This is not the case, for example, when you keep the temperature constant, since then heat must flow from the environment to your system (or vice versa) for $T$ to remain fixed.
But let's say the system you are interested in is small compared to the environment. Keeping track after all the thermodynamics of the surroundings is difficult, so we want to somehow treat the system alone without having to think all the time on what is happening outside. The way to do it, in the case of thermal contact only, is to define the Helmholtz free energy
$$F=U-TS$$
Here $U$ is the energy of the system, $S$ is its entropy but $T$ is the temperature of the environment (and it is fixed since your system is so small so that practically it has no effect on it). When $F$ is minimized, it can be shown (see here) that the entropy of the system + the environment is maximized, which is the condition you would expect for a system in thermal equilibrium.
Similarly, if your system can only exchange volume with the environment, the quantity that is minimized is known at the enthalpy
$$H=U+PV$$
In the case of both heat and volume exchange, you would use the Gibbs free energy
$$G=U-TS+PV$$
There is also a mathematical-inclined argument for this. You know that
$$rm dF=-Srm dT-Prm dV$$
so the natural variables of $F$ are $T$ and $V$, i.e. $F=Fleft(T,Vright)$. It means that $F$ is the right function to use if you can control both $T$ and $V$, which is the case for a system of constant volume in contact with a thermal bath. In the other cases
$$rm dH=Trm dS+Vrm dPLongrightarrow H=Hleft(S,Pright)$$
and
$$rm dG=-Srm dT+Vrm dPLongrightarrow G=Gleft(T,Pright)$$
You can clearly see which variables you can control and consequently which situations each thermodynamic potential fits. This mathematical trick of changing the variables of your function is known as the Legendre transformation. This is exactly the same as the relation between Lagrangians and Hamiltonians in classical mechanics.
To finish with an example, when treating liquid-gas phase transitions, it is customary to use the Gibbs free energy. This is the right thermodynamic potential since your system can exchange both its entropy $S$ and volume $V$ with the outside, such that the variables you control (the environment) are the corresponding conjugate variables - the temperature $T$ and the pressure $P$ respectively.
edited May 16 at 19:56
answered May 16 at 19:29
eranrecheseranreches
3,39311027
3,39311027
$begingroup$
So $F$ is $U$ disregarding the effects of heat transferred to it by the environment? So what energy left over is the 'free' energy? And the other potentials are $U$ but accounting for the effects the environment will have on its internal energy?
$endgroup$
– sangstar
May 16 at 19:37
$begingroup$
Yes, and one can show that for a reversible process $W=-Delta F$, which is why $F$ is the free energy - what you can actually put into work.
$endgroup$
– eranreches
May 16 at 19:43
$begingroup$
Why when I've worked out the work done due to isothermal processes in the past, I was fine to work with internal energy? Shouldn't I be working in Helmholtz free energy since processes are isothermal?
$endgroup$
– sangstar
May 16 at 20:50
$begingroup$
To be pedantic, the IUPAC convections on the names for Helmholtz and Gibbs energies do not include the word "free", and the Helmholtz energy is $A$ not $F$.
$endgroup$
– Jeffrey J Weimer
May 16 at 20:53
$begingroup$
@sangstar The relation $rm dU=Trm dS-Prm dV$ where $rm dW=Prm dV$ is still valid, so you can indeed use $U$ to get the work. But note: the change is energy is not simply the work as in mechanics, but contains another term due to entropy exchange with the surroundings. The change in $F$ contains just the work for reversible process. In addition, $U$ is not minimized so $rm dU$ is non-zero in equilibrium.
$endgroup$
– eranreches
May 16 at 21:13
add a comment |
$begingroup$
So $F$ is $U$ disregarding the effects of heat transferred to it by the environment? So what energy left over is the 'free' energy? And the other potentials are $U$ but accounting for the effects the environment will have on its internal energy?
$endgroup$
– sangstar
May 16 at 19:37
$begingroup$
Yes, and one can show that for a reversible process $W=-Delta F$, which is why $F$ is the free energy - what you can actually put into work.
$endgroup$
– eranreches
May 16 at 19:43
$begingroup$
Why when I've worked out the work done due to isothermal processes in the past, I was fine to work with internal energy? Shouldn't I be working in Helmholtz free energy since processes are isothermal?
$endgroup$
– sangstar
May 16 at 20:50
$begingroup$
To be pedantic, the IUPAC convections on the names for Helmholtz and Gibbs energies do not include the word "free", and the Helmholtz energy is $A$ not $F$.
$endgroup$
– Jeffrey J Weimer
May 16 at 20:53
$begingroup$
@sangstar The relation $rm dU=Trm dS-Prm dV$ where $rm dW=Prm dV$ is still valid, so you can indeed use $U$ to get the work. But note: the change is energy is not simply the work as in mechanics, but contains another term due to entropy exchange with the surroundings. The change in $F$ contains just the work for reversible process. In addition, $U$ is not minimized so $rm dU$ is non-zero in equilibrium.
$endgroup$
– eranreches
May 16 at 21:13
$begingroup$
So $F$ is $U$ disregarding the effects of heat transferred to it by the environment? So what energy left over is the 'free' energy? And the other potentials are $U$ but accounting for the effects the environment will have on its internal energy?
$endgroup$
– sangstar
May 16 at 19:37
$begingroup$
So $F$ is $U$ disregarding the effects of heat transferred to it by the environment? So what energy left over is the 'free' energy? And the other potentials are $U$ but accounting for the effects the environment will have on its internal energy?
$endgroup$
– sangstar
May 16 at 19:37
$begingroup$
Yes, and one can show that for a reversible process $W=-Delta F$, which is why $F$ is the free energy - what you can actually put into work.
$endgroup$
– eranreches
May 16 at 19:43
$begingroup$
Yes, and one can show that for a reversible process $W=-Delta F$, which is why $F$ is the free energy - what you can actually put into work.
$endgroup$
– eranreches
May 16 at 19:43
$begingroup$
Why when I've worked out the work done due to isothermal processes in the past, I was fine to work with internal energy? Shouldn't I be working in Helmholtz free energy since processes are isothermal?
$endgroup$
– sangstar
May 16 at 20:50
$begingroup$
Why when I've worked out the work done due to isothermal processes in the past, I was fine to work with internal energy? Shouldn't I be working in Helmholtz free energy since processes are isothermal?
$endgroup$
– sangstar
May 16 at 20:50
$begingroup$
To be pedantic, the IUPAC convections on the names for Helmholtz and Gibbs energies do not include the word "free", and the Helmholtz energy is $A$ not $F$.
$endgroup$
– Jeffrey J Weimer
May 16 at 20:53
$begingroup$
To be pedantic, the IUPAC convections on the names for Helmholtz and Gibbs energies do not include the word "free", and the Helmholtz energy is $A$ not $F$.
$endgroup$
– Jeffrey J Weimer
May 16 at 20:53
$begingroup$
@sangstar The relation $rm dU=Trm dS-Prm dV$ where $rm dW=Prm dV$ is still valid, so you can indeed use $U$ to get the work. But note: the change is energy is not simply the work as in mechanics, but contains another term due to entropy exchange with the surroundings. The change in $F$ contains just the work for reversible process. In addition, $U$ is not minimized so $rm dU$ is non-zero in equilibrium.
$endgroup$
– eranreches
May 16 at 21:13
$begingroup$
@sangstar The relation $rm dU=Trm dS-Prm dV$ where $rm dW=Prm dV$ is still valid, so you can indeed use $U$ to get the work. But note: the change is energy is not simply the work as in mechanics, but contains another term due to entropy exchange with the surroundings. The change in $F$ contains just the work for reversible process. In addition, $U$ is not minimized so $rm dU$ is non-zero in equilibrium.
$endgroup$
– eranreches
May 16 at 21:13
add a comment |
Thanks for contributing an answer to Physics Stack Exchange!
- Please be sure to answer the question. Provide details and share your research!
But avoid …
- Asking for help, clarification, or responding to other answers.
- Making statements based on opinion; back them up with references or personal experience.
Use MathJax to format equations. MathJax reference.
To learn more, see our tips on writing great answers.
Sign up or log in
StackExchange.ready(function ()
StackExchange.helpers.onClickDraftSave('#login-link');
);
Sign up using Google
Sign up using Facebook
Sign up using Email and Password
Post as a guest
Required, but never shown
StackExchange.ready(
function ()
StackExchange.openid.initPostLogin('.new-post-login', 'https%3a%2f%2fphysics.stackexchange.com%2fquestions%2f480488%2fwhy-is-choosing-a-suitable-thermodynamic-potential-important%23new-answer', 'question_page');
);
Post as a guest
Required, but never shown
Sign up or log in
StackExchange.ready(function ()
StackExchange.helpers.onClickDraftSave('#login-link');
);
Sign up using Google
Sign up using Facebook
Sign up using Email and Password
Post as a guest
Required, but never shown
Sign up or log in
StackExchange.ready(function ()
StackExchange.helpers.onClickDraftSave('#login-link');
);
Sign up using Google
Sign up using Facebook
Sign up using Email and Password
Post as a guest
Required, but never shown
Sign up or log in
StackExchange.ready(function ()
StackExchange.helpers.onClickDraftSave('#login-link');
);
Sign up using Google
Sign up using Facebook
Sign up using Email and Password
Sign up using Google
Sign up using Facebook
Sign up using Email and Password
Post as a guest
Required, but never shown
Required, but never shown
Required, but never shown
Required, but never shown
Required, but never shown
Required, but never shown
Required, but never shown
Required, but never shown
Required, but never shown
PMDoSWzEgs8wx GF,pI83s2XqVETDmFKEup vpXmvqTHyl,MK g,BMK,gu,3i4WkVQ6u yYOM8
$begingroup$
what do you mean by what do we care? If you use the energy instead of the Free-energy for an isothermal process then it is incorrect.
$endgroup$
– lakehal
May 16 at 19:26
1
$begingroup$
Incorrect how? I've never actually used the free energies before, and only know them in terms of how they're defined so that's why it's not clear to me.
$endgroup$
– sangstar
May 16 at 19:27
1
$begingroup$
@lakehal What are you talking about? There is no rule against "using energy instead of free-energy" for an isothermal process, you simply need to take account for the constant temperature in the rest of your analysis.
$endgroup$
– Drew
May 16 at 20:52
$begingroup$
@Drew but it is not minimised.
$endgroup$
– lakehal
May 16 at 21:25